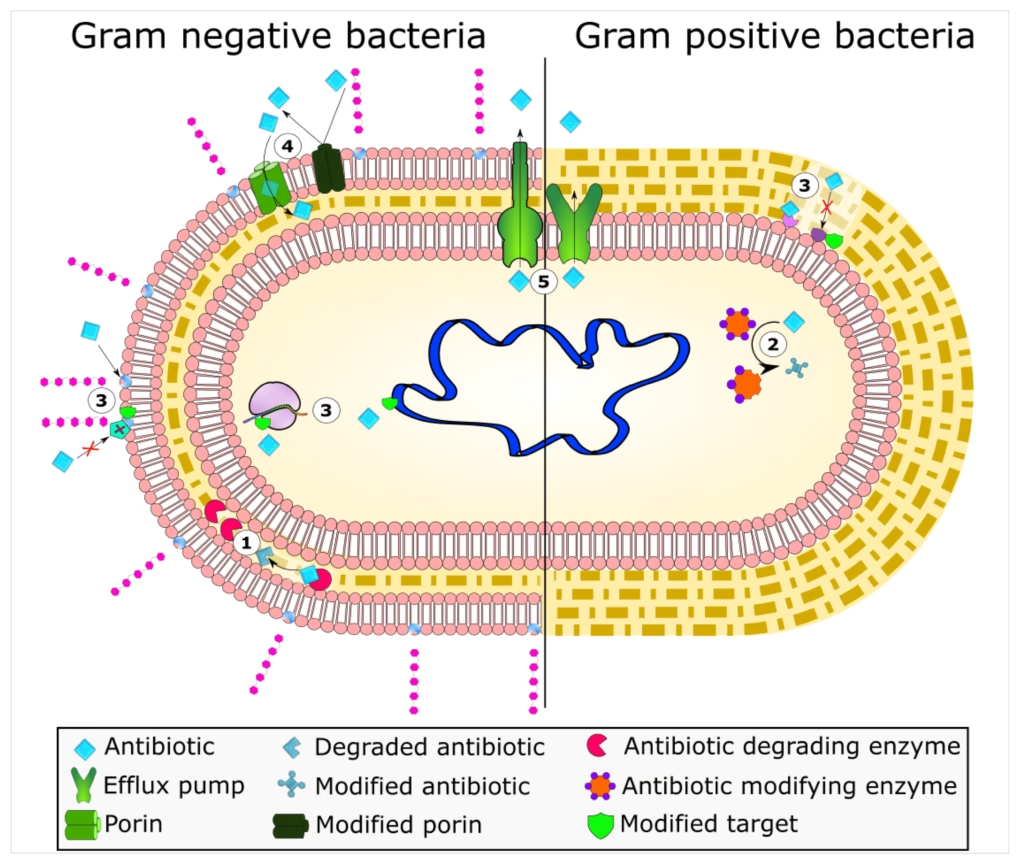
Antibiotics are drugs used to treat bacterial infections. They target specific bacterial structures or processes essential for bacterial survival and replication. Here’s an overview of the principles and mechanisms of action of antibiotics:
Principles of Antibiotics
-
Selective Toxicity:
- Antibiotics are designed to target features unique to bacteria, sparing human cells specifically. This is possible because bacterial cells are structurally and functionally different from human cells. For example, bacteria have cell walls, which human cells lack. By targeting these distinct structures, antibiotics minimize harm to the human host.
-
Spectrum of Activity:
- Broad-Spectrum Antibiotics: Effective against a wide range of bacteria, including both Gram-positive and Gram-negative species. Examples include tetracyclines, chloramphenicol, and some penicillins. Broad-spectrum antibiotics are often used when specific causative bacteria are unknown. However, they can also disrupt normal microbiota, potentially leading to secondary infections (e.g., C. difficile colitis).
- Narrow-Spectrum Antibiotics: Effective against specific types of bacteria, such as Gram-positive or Gram-negative only. Examples include vancomycin (mainly for Gram-positive bacteria) and colistin (mainly for Gram-negative bacteria). Narrow-spectrum antibiotics are typically preferred when the causative bacteria are identified, as they reduce the impact on beneficial bacteria and minimize resistance development.
-
Bactericidal vs. Bacteriostatic:
- Bactericidal Antibiotics kill bacteria directly. These are typically used in severe infections where the immune system might need additional help to eradicate bacteria quickly. Examples include penicillins and cephalosporins.
- Bacteriostatic Antibiotics inhibit bacterial growth, slowing bacterial replication and allowing the host’s immune system to clear the infection. Examples include tetracyclines and macrolides.
Modes of Action of Antibiotics
Antibiotics target specific bacterial structures or functions essential for survival. Below are the main mechanisms of action with additional details and examples:
A. Inhibition of Cell Wall Synthesis
- Mechanism: Many bacteria, particularly Gram-positive bacteria, have a cell wall composed of peptidoglycan, which provides structural integrity and protection. Antibiotics that inhibit cell wall synthesis prevent the formation of peptidoglycan, making bacterial cells more prone to osmotic lysis.
- Examples:
- Penicillins and Cephalosporins: These are β-lactam antibiotics. They bind to penicillin-binding proteins (PBPs), enzymes in the final stages of peptidoglycan synthesis. By inhibiting PBPs, they prevent cell wall cross-linking, leading to bacterial cell rupture.
- Vancomycin: This antibiotic binds to D-Ala-D-Ala termini of peptidoglycan precursors, blocking cell wall synthesis in Gram-positive bacteria. It’s often reserved for MRSA (methicillin-resistant Staphylococcus aureus) and other resistant strains.
- Bacitracin: This antibiotic inhibits the dephosphorylation of bactoprenol, a carrier molecule that transports peptidoglycan precursors across the cell membrane.
B. Inhibition of Protein Synthesis
- Mechanism: Bacterial ribosomes are 70S (composed of 30S and 50S subunits), while human ribosomes are 80S. This difference allows antibiotics to target bacterial protein synthesis selectively.
- Examples:
- Aminoglycosides (e.g., gentamicin, streptomycin) Bind irreversibly to the 30S subunit, causing mRNA misreading and synthesis of faulty proteins. These are bactericidal antibiotics.
- Tetracyclines Bind to the 30S subunit and block the attachment of tRNA, preventing the addition of new amino acids to the growing polypeptide chain. Tetracyclines are bacteriostatic.
- Macrolides (e.g., erythromycin, azithromycin) Bind to the 50S subunit and inhibit translocation, the ribosome’s movement along the mRNA. Macrolides are bacteriostatic.
- Chloramphenicol Binds to the 50S subunit and inhibits peptidyl transferase, an enzyme necessary for peptide bond formation.
C. Inhibition of Nucleic Acid Synthesis
- Mechanism: These antibiotics disrupt bacterial DNA or RNA synthesis processes essential for cell replication and function.
- Examples:
- Quinolones (e.g., ciprofloxacin, levofloxacin): Inhibit DNA gyrase and topoisomerase IV, enzymes required for DNA replication and supercoiling. Quinolones prevent bacterial DNA from unwinding and replicating by interfering with these enzymes.
- Rifamycins (e.g., rifampin): Bind to bacterial RNA polymerase, inhibiting transcription. Rifampin is used to treat tuberculosis, often in combination with other antibiotics to prevent resistance.
- Metronidazole: Generates free radicals that cause DNA strand breakage, specifically effective against anaerobic bacteria and certain protozoa.
D. Disruption of Cell Membrane Function
- Mechanism: These antibiotics interact with bacterial membranes, causing leakage of essential cellular components.
- Examples:
- Polymyxins (e.g., polymyxin B and colistin): These antibiotics interact with the phospholipids in the cell membrane of Gram-negative bacteria, causing increased permeability and cell death. Polymyxins are often used as a last resort for multidrug-resistant infections.
- Daptomycin: This antibiotic inserts into the cell membrane of Gram-positive bacteria, disrupting membrane potential and leading to cell death.
E. Inhibition of Metabolic Pathways
- Mechanism: Some antibiotics target essential bacterial metabolic pathways, such as folic acid synthesis, which is necessary for DNA synthesis and repair.
- Examples:
- Sulfonamides: Inhibit the enzyme dihydropteroate synthase, blocking the synthesis of dihydrofolic acid, a precursor to folic acid.
- Trimethoprim: Inhibits dihydrofolate reductase, another enzyme in the folic acid synthesis pathway. Sulfonamides and trimethoprim are often used in a synergistic combination called co-trimoxazole (TMP-SMX).
Antibiotic Resistance
Antibiotic resistance is a growing concern, resulting from bacterial adaptations that neutralize or evade antibiotics. Mechanisms include:
- Enzymatic Degradation or Modification:
- Bacteria produce enzymes that inactivate antibiotics. For example, β-lactamases break down the β-lactam ring in penicillins and cephalosporins, rendering them ineffective.
- Some bacteria also produce aminoglycoside-modifying enzymes that alter aminoglycosides, reducing their effectiveness.
- Alteration of Target Sites:
- Bacteria can alter the molecular target of an antibiotic, reducing the drug’s ability to bind effectively.
- Example: MRSA alters its PBPs, which reduces the binding of β-lactam antibiotics.
- Efflux Pumps:
- Bacteria use efflux pumps to actively transport antibiotics out of the cell before they reach effective concentrations.
- Efflux pumps contribute to resistance to tetracyclines, fluoroquinolones, and macrolides.
- Reduced Permeability:
- Some bacteria decrease the permeability of their cell wall or membrane, limiting antibiotic entry.
- Gram-negative bacteria can be less permeable to certain antibiotics (e.g., vancomycin) with their outer membrane.
- Bypass of Metabolic Pathways:
- Some bacteria develop alternative metabolic pathways that bypass the blocked step in folic acid synthesis affected by sulfonamides and trimethoprim.
Chemotherapeutic Agents for Bacterial Infections
Bacterial chemotherapeutic agents (antibiotics) can be classified based on their target mechanisms:
a. Cell Wall Synthesis Inhibitors
These agents interfere with the formation of the bacterial cell wall, leading to cell lysis and death.
- Penicillins (e.g., amoxicillin, penicillin G): Bind to penicillin-binding proteins (PBPs) and inhibit peptidoglycan cross-linking, essential for cell wall integrity.
- Cephalosporins (e.g., ceftriaxone, cefuroxime): Similar to penicillins but with a broader range of activity and more resistance to β-lactamases.
- Carbapenems (e.g., imipenem, meropenem): Broad-spectrum β-lactams used for multi-drug-resistant infections.
- Vancomycin: Targets D-Ala-D-Ala residues in peptidoglycan, primarily for Gram-positive bacteria like MRSA.
b. Protein Synthesis Inhibitors
These antibiotics target bacterial ribosomes (70S) and interfere with protein synthesis.
- Aminoglycosides (e.g., gentamicin, streptomycin) Bind to the 30S ribosomal subunit and cause misreading of mRNA, leading to defective proteins.
- Tetracyclines (e.g., doxycycline, minocycline) Bind to the 30S subunit and block the attachment of tRNA, halting protein synthesis.
- Macrolides (e.g., erythromycin, azithromycin) Bind to the 50S subunit and inhibit translocation during protein elongation.
- Chloramphenicol: Inhibits peptidyl transferase on the 50S subunit, blocking peptide bond formation.
c. Nucleic Acid Synthesis Inhibitors
These agents disrupt bacterial DNA or RNA synthesis.
- Fluoroquinolones (e.g., ciprofloxacin, levofloxacin): Inhibit DNA gyrase and topoisomerase IV, preventing DNA replication.
- Rifamycins (e.g., rifampin): Inhibit bacterial RNA polymerase, blocking transcription, used in tuberculosis treatment.
d. Cell Membrane Disruptors
These agents increase membrane permeability, leading to cell death.
- Polymyxins (e.g., polymyxin B, colistin): Target the lipopolysaccharide layer in Gram-negative bacteria, disrupting cell membranes.
- Daptomycin Targets Gram-positive bacteria by disrupting the cell membrane, causing depolarization and cell death.
e. Metabolic Pathway Inhibitors
These drugs inhibit essential bacterial metabolic pathways, such as folic acid synthesis.
- Sulfonamides (e.g., sulfamethoxazole): Compete with PABA and inhibit dihydropteroate synthase, a critical enzyme in folic acid synthesis.
- Trimethoprim: Inhibits dihydrofolate reductase, another enzyme in the folic acid synthesis pathway, often combined with sulfonamides (e.g., TMP-SMX).
Chemotherapeutic Agents for Fungal Infections
Fungal infections (mycoses) can be superficial or systemic, and antifungal agents are used to treat them. These agents target specific components of fungal cells, especially the cell membrane and cell wall.
a. Cell Membrane Disruptors (Ergosterol Inhibitors)
Fungal cell membranes contain ergosterol (instead of cholesterol, found in human cells). Many antifungals target ergosterol or its synthesis.
- Polyenes (e.g., amphotericin B, nystatin) Bind directly to ergosterol in the fungal cell membrane, forming pores and causing cell leakage and death. Amphotericin B is used for severe systemic infections.
- Azoles (e.g., fluconazole, itraconazole, ketoconazole): Inhibit ergosterol synthesis by blocking the enzyme 14α-demethylase, essential for converting lanosterol to ergosterol. Azoles are used to treat a range of superficial and systemic fungal infections.
- Allylamines (e.g., terbinafine): Inhibit squalene epoxidase, another enzyme in the ergosterol biosynthesis pathway, causing toxic squalene accumulation in fungal cells.
b. Cell Wall Synthesis Inhibitors
Fungal cell walls contain β-glucans, which are not present in human cells. Agents targeting this pathway are specific to fungi.
- Echinocandins (e.g., caspofungin, micafungin, anidulafungin): Inhibit β-1,3-glucan synthase, an enzyme required for β-glucan synthesis, weakening the cell wall and leading to cell death. Echinocandins are effective against Candida and Aspergillus species.
c. Nucleic Acid Synthesis Inhibitors
Some antifungals interfere with DNA or RNA synthesis in fungi.
- Flucytosine (5-FC): A pyrimidine analog converted into 5-fluorouracil in fungal cells, interfering with DNA and RNA synthesis. It’s often used in combination with amphotericin B for cryptococcal infections.
d. Mitotic Inhibitors
These drugs interfere with fungal cell division.
- Griseofulvin: Binds to tubulin, disrupting microtubule formation and inhibiting fungal cell mitosis. Primarily used to treat dermatophyte infections like ringworm.
Challenges and Resistance in Chemotherapeutic Agents
- Bacterial Resistance: Resistance to antibiotics can develop through mechanisms like enzymatic degradation (e.g., β-lactamases), alteration of target sites, efflux pumps, and metabolic pathway changes. Examples include methicillin-resistant Staphylococcus aureus (MRSA) and multidrug-resistant Pseudomonas aeruginosa.
- Fungal Resistance: Resistance to antifungal agents, particularly in species like Candida and Aspergillus, is also a growing concern. Mechanisms include mutations in the target enzyme, increased efflux pump activity, and biofilm formation, which can shield fungi from antifungal action.